Boron and Photocatalysis
The increasing reliance on renewable energy sources has led to many studies and research into boron and photocatalysis for applications ranging from solar energy, pollutant removal, artificial photosynthesis, energy storage, and fuel evolution.
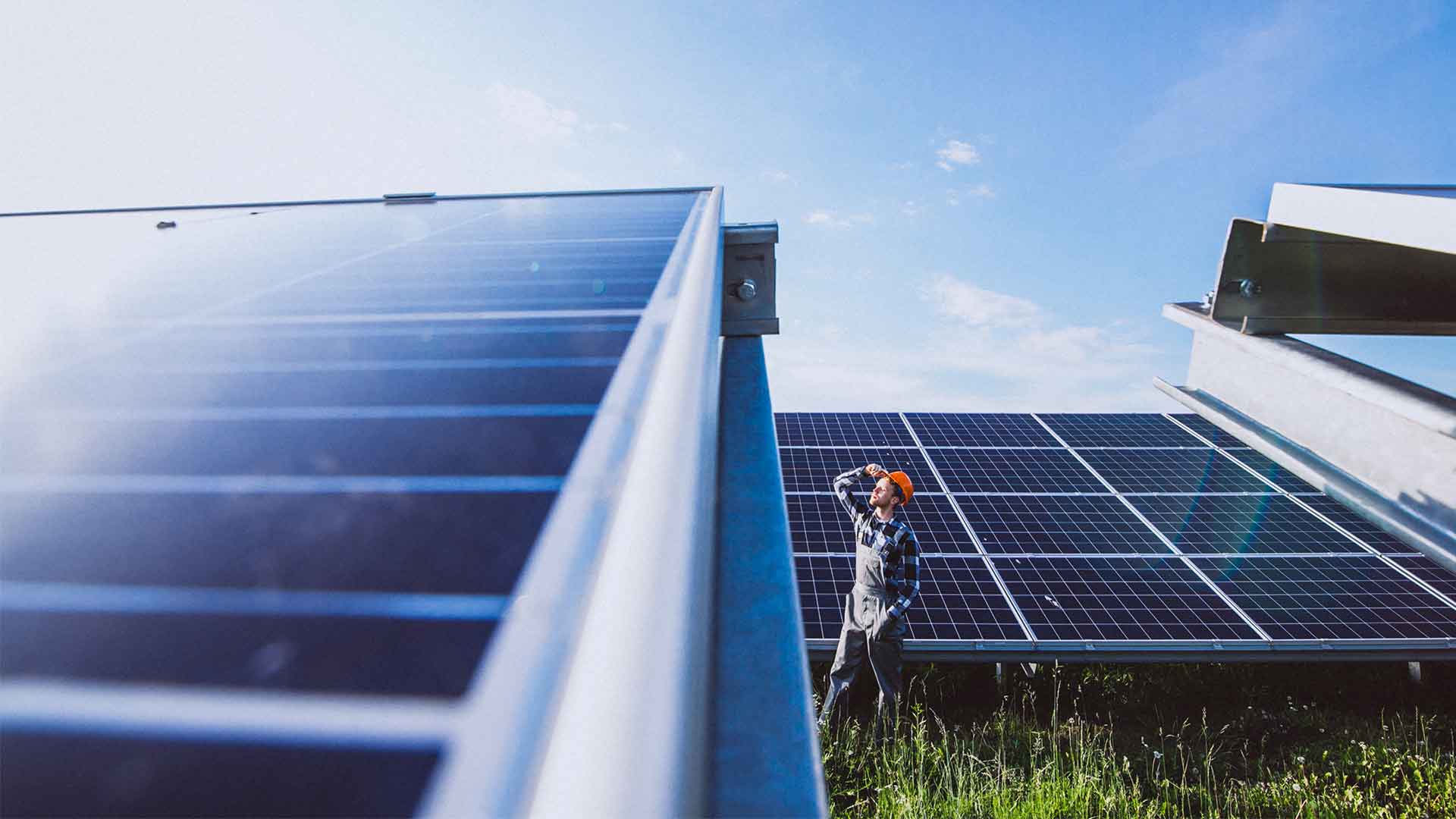
Boron and Photocatalysis
New technologies are being rapidly developed and deployed. For example, boron-based magnesium tribromide MXene Nanosheets could be used for next-generation photocatalysis or energy storage applications. Boron can revolutionize solar energy when mixed with other elements because of its excellent conductivity and photocatalytic activities.
Photocatalysis and Boron
The climate crisis has pushed countries worldwide to opt for renewable sources of energy. These technologies are being rapidly developed and deployed to assist governments in meeting their net-zero carbon emission targets by 2050. Multiple industries are benefiting from research into advanced materials.
One such example is boron-based magnesium tetrabromide MXene nanosheets which could be used in next-generation photocatalysis and energy storage applications. Several studies suggest that boron mixed with other elements can revolutionize the field of solar energy due to its capability of excellent conductivity and photocatalytic activity.
Choosing Renewable Energy
Since the industrial revolution, fossil fuels have been the primary source of human energy. But this has come with a price: rising temperatures have threatened the stability of planet ecosystems, vulnerable communities, and resource depletion has raised the possibility of future energy crises. The exploitation of fossil fuels also results in physical damage to ecosystems, pollution of water bodies due to industrial runoff, and environmental problems such as oil spillages.
Renewable energy is increasingly being used to replace fossil fuels like oil, gas, and coal. These technologies can help avert the climate crisis. Solar energy plays a crucial role, providing unlimited, clean energy to replace fossil fuels. The last decade has witnessed the development of new technologies and advanced materials in solar energy conversion, making it economically viable to harness the abundant sun’s energy.
Photocatalytic Solar Energy Conversion
Because of its potential to provide clean, renewable energy storage and environmental protection, this field of energy research is gaining a lot of attention. Photocatalysis converts sunlight into chemical energy and provides storage solutions. Photocatalytic solar power conversion is beneficial for green energy generation and has applications in fields like pollutant removal, artificial photosynthesis, and green fuel production. Photocatalysis improves energy conversion-related reactions like carbon dioxide reduction, light-driven water splitting, and nitrogen fixation.
Photocatalysts must have a conduction band bottom that is more negative than the reduction potential for hydrogen to allow the production of H2 molecules. Different teams have researched advanced materials that possess superior photocatalytic properties to realize the full potential of this technology. One group is now investigating the possibility of using MXene-like nanomaterials that are boron-based.
In November 2021, a paper was published online that explored the potential use of these nano-scaled 2D materials as photocatalysts. MXenes can be made up of transition metal carbides, carbonitrides, or nitrides. By etching specific atomic layers of precursor materials, they are prepared. MXenes have unique electronic and structural properties, appealing for photocatalysis applications.
Hydrophilic functionalities of MXenes make them suitable for photocatalytic H2 synthesis from water. This is a potential method that allows full utilization of solar energy. These sites can be connected to other molecules, such as water, dyes, metals, and even semiconductors, which gives MXenes unusual reactive activities.
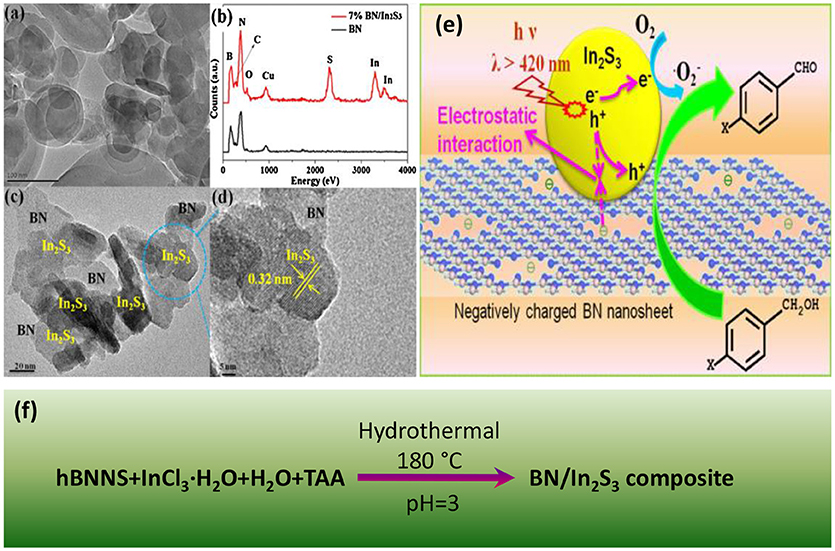
Boron Photocatalysis
Source: https://www.frontiersin.org/articles/10.3389/fchem.2018.00440/full
Boron in Photocatalysis
The research paper focuses on boron-based magnesium Tetraboride (MgB4) MXene nanosheets that can be used as photocatalysts. The study used standard MXene preparation methods. To create the new material, a single Mg layer was made from magnesium dibromide MgB2. An aqueous solution of hydrochloric acid was used as the etching agent. The team examined the material’s optical, chemical, and structural characteristics.
MXenes with metal layers transfer and separate photogenerated carriers. They also harvest higher levels of photonics which results in more active electron-hole pairs. Magnesium tetrabromide’s boron and metal bonds give it superior mechanical, thermal, and chemical stability, as well as high levels of thermal and electrical conductivity. The study revealed that the nanosheets were capable of photocatalysis of H2 from water molecules using stable and efficient results. The nanosheets have many exposed metal sites and superior conductivity. It was found that the material had superior solar energy harvesting and absorbing capabilities.
They prepared MgB4 MXene like nanosheets using layered MgB2 with the same procedure as for MXene preparation starting from the MAX phase. MgB4 nanosheets, as obtained, have high stable photocatalytic activities. This is due to many exposed terminal metal sites on the surface, excellent metallic layer conductivity, higher solar energy absorption and harvesting abilities, and a lot more. These findings show the immense potential of MgB4 photocatalysis. They may also open up new avenues to develop more efficient boron photocatalysts that can be used for everything from pollutant removal to artificial photosynthesis and fuel evolution.
Further, a study of highly-dispersed graphene nanoribbons with boron-doped graphene revealed improved conductibility and photocatalysis. B-GNRs, prepared using a simple vacuum activation process, showed p-type semiconductor characteristics and had many more zigzag and armchair edges to control the bandgap. B-GNRs were used to photodegrade Rhodamine B and demonstrated excellent conductivity and photocatalytic activity.
Researchers also discovered the photocatalytic ability of the boron-induced Ti3+ compounds in the TiO2 surface layer. Interstitial B was introduced to create and stabilize sufficient Ti3+ species in TiO’s surface layer. This is a new and straightforward approach. Both experimental and computational data demonstrated that interstitial B could generate stable and abundant Ti3+ species and create structural disorder in B-TiO photocatalysts’ surface layer. These structural changes result in significant variations in the density of their states. This is when two new groups of middle-gap states are created. It also results in a considerable decrease in the bandgap.
This bandgap engineering can promote photogenerated electron mobility and provide shallow trapping spots (B-O–Ti3+) for photogenerated carriers to stop them from rapid recombination. This eventually improves the sunlight photocatalytic activities of B-TiO towards hydrogen production and water splitting. These experimental and theoretical calculations allowed for a better understanding at the atomic level of the photocatalytic process. They will also help to design highly efficient titania-based photocatalysts rationally.
A study was done on a water-stable boron-doped boron nitride photocatalyst that can be used for visible-light-driven H2 evolution and CO2 reduction. This research opens up the possibility of expanding the BN photocatalyst platform for a wider variety of reactions. With the long-term goal of integrated photocatalytic systems, it is crucial to develop robust, multifunctional photocatalysts capable of facilitating hydrogen evolution via photoreforming water and gas-phase CO2 photoreduction.
They presented the boron-doped boron oxynitride, an addition to the photocatalytic platform of boron (BN), capable of fulfilling this goal. EPR studies showed hyperfine interactions between the free charges at discrete OB3 sites. These sites displayed out-of-plane symmetry and nuclei from neighboring boron atoms. This material solves two long-standing problems associated with BN-based materials: instabilities in water and lack of photoactivity under visible lighting. B-BNO can be used to facilitate liquid phase H2 transformation and gas-phase CO2 photoreduction using UV-Vis or deep visible radiation without the use of any co-catalysts. Both reactions with B-BNO have higher evolution rates, quantum yields, and selectivities than their porous BNO counterparts P25 TiO2 or bulk g-C3N4.
All the studies and research open the door to more efficient catalysts made from boron for energy storage and conversion.