In today’s podcast, we’re going to look at the science of solar energy with solar cells. A solar cell, also called a photovoltaic cell or PV, is the basic building block of a solar power system. It’s made up of semiconductor materials that convert sunlight into electricity. But how does it all work? And what role does boron play in solar cells?
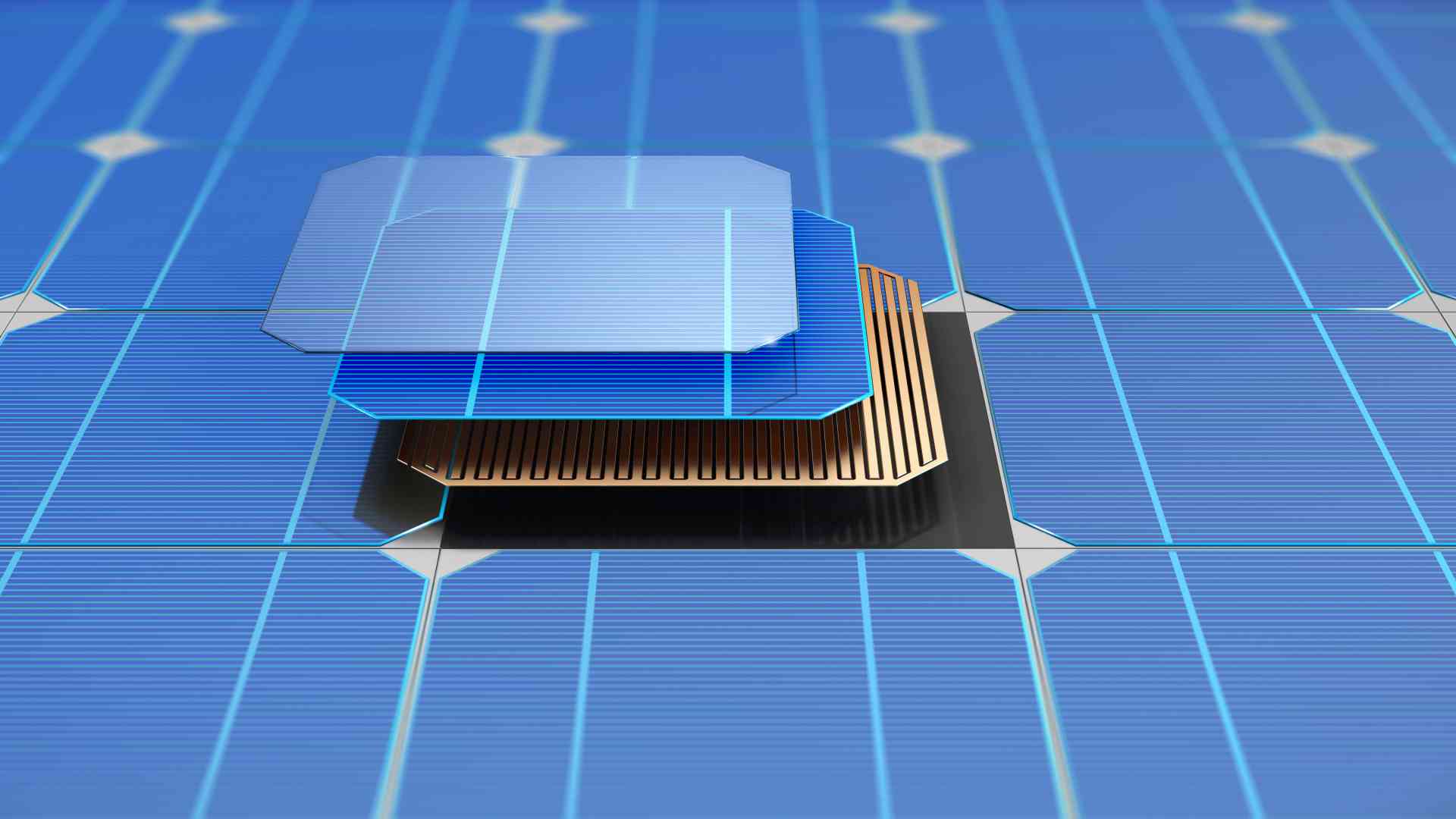
Solar Cell
The Science of Solar Energy with Solar Cells
Let’s start by looking at photovoltaic technology. Photovoltaics derives its name from the photovoltaic effect, which converts light (photons) to electricity (voltage). In 1954. Bell laboratory scientists first utilized this effect and built a working silicon solar cell that produced an electric current when sunlight strikes. Solar cells were then used to power space satellites and small appliances like calculators and watches. Solar electricity is highly cost-competitive in many regions and photovoltaics are being deployed on massive scales to support the electricity system.
The performance of a photovoltaic cell is simply the amount of electrical power produced by the cell to the energy produced by the light shining on it, indicating the sales effectiveness in converting energy from one form to another.
The amount of power generated from these photovoltaic cells is influenced by the characteristics of the available light, intensity, and wavelength, and the cells’ multiple performance attributes. The bandgap of PV semiconductors is an essential property of deciding the light wavelength the material can absorb and convert to electrical energy. If the semiconductor’s band gap meets the light wavelength shining on the PV cell, the cell can effectively use all the available power.
Let’s look at the types of solar cells in production. Silicon is the most commonly used semiconductor material in solar cells, accounting for approximately 95% of panels sold to date. It’s the second, most abundant element on the planet after oxygen and the most widely used semiconductor in computer chips. Crystalline silicon cells are built from silicon atoms linked together to form a crystal lattice. This lattice creates an organized structure that improves the efficiency of lights to electricity conversion.
Silicon solar cells currently offer high efficiency, low cost, and long lifetime. Devices are expected to last for 25 years or more. While producing over 80% of the original power.
A second type of solar cell besides silicon solar cells is the thin-film solar cell. Thin-film solar cells are produced from a very thin layer of semiconductor material such as cadmium, telluride, or copper Indian gallium diselenide. The thickness of such cell layers is only a few micrometers or several millions of a meter.
These cells are flexible and lightweight, hence ideal for portable applications, as issued in the soldier’s backpack or in other products that generate electricity from the sun such as windows. Additionally, some types of thin-film solar cells benefit from advanced manufacturing activities that require low energy and are easy to expand.
A third type of solar cell is the organic solar cell. Organic solar cells are made of carbon-rich. organic compounds to improve a specific PV cell function such as band gap, transparency, or color. OPV cells, as they’re known, are only about half as proficient as silicon solar cells and have shorter processing lifetimes. But they are cheaper to produce in large quantities. They can also be applied to flexible plastics or other supporting materials allowing OPV to be used for many applications.
The last type of solar cell in this discussion is the concentrated solar cell. Concentrated photovoltaics, CPV, is a solar technology that uses lenses or mirrors to direct large amounts of sunlight onto small, highly efficient solar cells. Hence, they require less PV material to capture the same amount of sunlight.
However, CPV systems are more expensive to produce and install. And they require special tracking devices to track the sun’s movement during the day. As a result, the CPV industry has found it difficult to demonstrate a cost advantage over conventional solar panels.
Let’s turn to looking at how solar cells work. A solar cell typically consists of two layers of Silicon; one P-type and one N-type. In the N-type layer, there’s an excess of electrons while in the P-type layer, there’s an excess of positively charged holes. At the junction between the two layers, electrons from the N-type side, flow into the holes on the P-type side. This creates a depletion zone around the junction where electrons fill the holes. When all of the holes in the depletion zone are filled with electrons. The P-type side of the depletion zone now has negatively charged ions. And the N-type side has positively charged ions.
The occurrence of these oppositely charged ions generates an internal electric field, which prevents electrons from the N-type layer from filling holes in the P-type there. When sunlight falls on a solar cell, electrons are ejected, resulting in the formation of holes – the voids left by the escaping electrons.
If this occurs in an electric field, the electrons will move to the N-type layer. And the holes will move to the P-type layer. After connecting the N-type and P-type layers with a metallic wire, electrons flow from the N-type to the P-type layer by passing the depletion zone and then go through the exterior wire back to the entire layer, generating electricity flow.
P-type silicon is created by atoms that have one less electron in a higher energy level than Silicon such as boron or gallium. As boron has one less electron required to form the bonds with the surrounding Silicon atoms, a hole is formed.
In comparison, N-type silicon is created by combining atoms with one extra electron at a higher energy level than Silicon, such as phosphorus.
What about boron and its contribution to solar cell productivity? Boron is used in solar cells as an anti-reflection coating primarily. Anti-reflection coatings reduce losses from incident sunlight that does not pass through or mix during the manufacturing process. This allows for better electrical, conductivity and increased efficiency.
In recent years, boron’s potential for use in energy storage has also been explored. Solar energy is a renewable resource that can be used to generate electricity. However, the current methods for transferring solar energy from areas of high productivity to industrialized areas result in significant energy losses.
Boron could potentially be used to reduce or eliminate these losses. Boron is a light element with a high affinity for electrons. This makes it ideal for use in electrical storage devices such as batteries. If used in this way, boron improves the efficiency of solar energy systems by reducing or eliminating the need for power lines and other infrastructure, which results in energy losses.
Additionally, boron is non-toxic and fairly abundant in the Earth’s crust making it a safe and sustainable option for energy storage in the future.
And that’s all from Borates Today. For more information on solar cells and the science behind solar energy and the contribution of boron, please refer to Borates Today, website. Meanwhile, thanks for listening.