Advanced energy
Energy is the next big thing in an increasingly competitive market. The most important contributors to this are battery production, so it’s time for investors and analysts to get up-to-date on their latest trends.
Energy storage has been a key issue in recent years as we transition away from fossil fuels and towards clean renewable power sources. To store intermittent solar or wind power, we need some way of storing electricity when the sun isn’t shining or the wind isn’t blowing, which means investing heavily in batteries. Batteries help us bridge that gap between supply and demand by charging during low usage periods like nighttime or off-peak hours and then releasing stored energy during peak hours when we need it most.
The current advances in data-driven materials engineering demonstrate that the application of machine learning technology would significantly facilitate the design and development of advanced energy materials and enhance their discovery and deployment. In this article, the importance and necessity of developing new energy materials towards contributing to global carbon neutrality are presented.
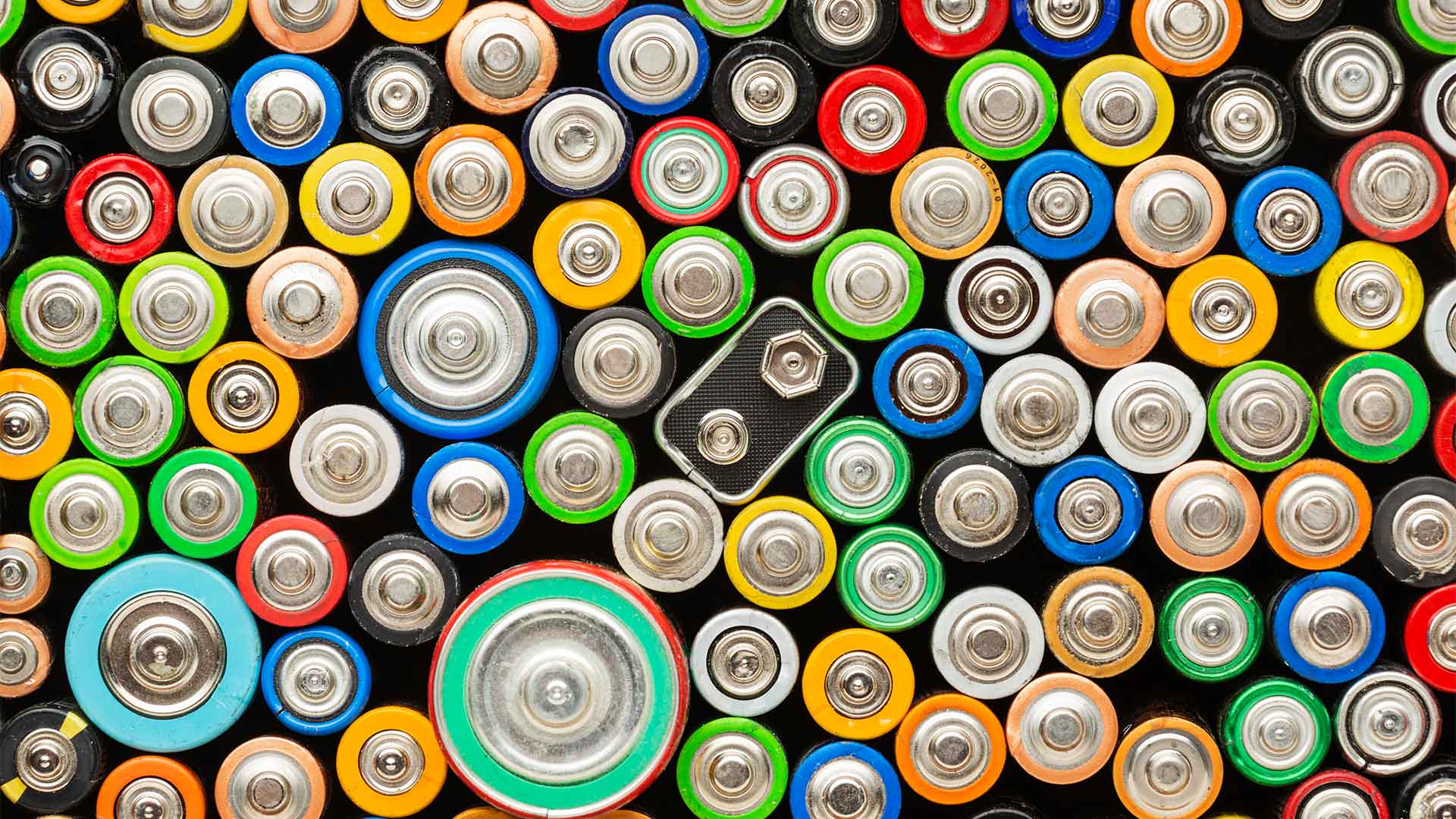
The Deal With Energy Storage
Energy storage is the capture of energy produced at one time for use at a later time to reduce imbalances between energy demand and energy production. A device that stores energy is generally called an accumulator battery. Energy comes in multiple forms including radiation, chemical gravitational potential electrical potential, electricity, elevated temperature, latent heat kinetic. Energy storage involves converting energy from forms that are difficult to store to more conveniently or economically storable forms. (en.wikipedia.org)
Some technologies provide short-term energy storage, while others can endure for much longer. Bulk energy storage is currently dominated by hydroelectric dams, both conventional as well as pumped.
Energy storage systems provide a wide array of technological approaches to managing our power supply to create a more resilient energy infrastructure and bring cost savings to utilities and consumers. To help understand the diverse approaches currently being deployed around the world, we have divided them into five main categories:
- Batteries – a range of electrochemical storage solutions, including advanced chemistry batteries, flow batteries, and capacitors
- Thermal – capturing heat and cold to create energy on-demand or offset energy needs
- Mechanical Storage – other innovative technologies to harness kinetic or gravitational energy to store electricity
- Hydrogen – excess electricity generation can be converted into hydrogen via electrolysis and stored
- Pumped Hydropower – creating large-scale reservoirs of energy with water
Batteries
The contributions of several scientists and innovators created our understanding of the forces of electricity, but Alessandro Volta is credited with the invention of the first battery in 1800. On its most basic level, a battery is a device consisting of one or more electrochemical cells that convert stored chemical energy into electrical energy.
Each cell contains a positive terminal, or cathode, and a negative terminal, or anode. Electrolytes allow ions to move between the electrodes and terminals, which allows current to flow out of the battery to perform work.
Advances in technology and materials have greatly increased the reliability, output, and density of modern battery systems, and economies of scale have dramatically reduced the associated cost. Continued innovation has created new technologies like electrochemical capacitors that can be charged and discharged simultaneously and instantly and provide an almost unlimited operational lifespan.
The Different Types Of Batteries We Should Know About
- Lithium-Ion (Li-Ion) Batteries
- Lead Batteries
- Redox Flow Batteries
- Vanadium Redox (VRB) Flow Batteries
- Nickel-Cadmium (NI-CD) Batteries
- Sodium Sulfur (NAS) Batteries
- Electrochemical Capacitors
- Iron-Chromium (ICB) Flow Batteries
- Zinc-Bromine (ZNBR) Flow Batteries
The Production Of Lithium-Ion Batteries Is Increasing At An Incredible Speed
After Exxon chemist Stanley Whittingham developed the concept of lithium-ion batteries in the 1970s, Sony and Asahi Kasei created the first commercial product in 1991. The first batteries were used for consumer electronics and now, building on the success of these Li-ion batteries, many companies are developing larger-format cells for use in energy-storage applications.
Many also expect there to be significant synergies with the emergence of electric vehicles (EVs) powered by Li-ion batteries. The flexibility of Li-ion technology in EV applications, from small high-power batteries for power buffering in hybrids, to medium-power batteries providing both electric-only range and power buffering in plug-in hybrids, to high-energy batteries in electric-only vehicles, has similar value in stationary energy storage.
Li-ion batteries have been deployed in a wide range of energy-storage applications, ranging from energy-type batteries of a few kilowatt-hours in residential systems with rooftop photovoltaic arrays to multi-megawatt containerized batteries for the provision of grid ancillary services. ( https://energystorage.org/why-energy-storage/technologies/solid-electrode-batteries/)
WORLD LITHIUM ION BATTERY PRODUCTION
As of Dec 2019, the number of lithium-ion battery mega factories in the pipeline has reached 115 plants. The world’s leading EV and battery manufacturer added a huge 564GWh of pipeline capacity in 2019 to a global total of 2068.3GWh or the equivalent of 40 million EVs by 2028.
In Jan 2019, Benchmark Minerals’ saw a Lithium-ion Battery Megafactory pipeline of 68 plants with a total capacity of 1.45TWh by 2028.
Europe’s planned 2018 lithium-ion cell battery capacity is now 348GWh. (energycentral.com)
China plans to add 564GWh by 2028 and has 88 of 115 lithium-ion battery mega factories in the pipeline to 2029.
Production technology for automotive lithium-ion battery (LIB) cells and packs has improved considerably in the past five years. However, the transfer of developments in materials, cell design and processes from lab scale to production scale remains a challenge due to a large number of consecutive process steps and the significant impact of material properties, electrode compositions and, cell designs on processes.
Perks Of Using Lithium-Ion Batteries
High Energy Density: One of the biggest advantages of a lithium-ion battery is its high energy density. To put it straight, lithium-ion batteries can last way longer between charges all the while maintaining a high current output. That makes it the perfect battery for most modern needs.
Low Self Discharge: Not only whilst being used, but lithium-ion batteries have a clear advantage when not being used as well. When kept idle, the rate of self-discharge, a common phenomenon in batteries, is extremely low. In fact, in most cases, it is as good as being negligent.
Low to Minimum Maintenance: Lithium-ion batteries are popular for their low maintenance batteries too. Most other cells like Nickel Cadmium batteries have a huge cost of ownership and maintenance.
Options: One of the biggest advantages of lithium-ion batteries is the fact that they come in all shapes and sizes- presenting users with a large number of options to choose from according to their needs.
The Role Of Boron(As Borates) In Batteries And Capacitors
The application of boron-based materials has been the key elements in commercial electrolytes for lithium-ion batteries and improved energy storage.
Borates lead to improvements in a variety of battery applications, including surface treatment of graphite anodes, catalyzing the synthesis of graphite, and as electrolyte additives.
Borate compounds, including lithium bis(oxalate)borate (LiBOB), are widely used in commercial lithium-Ion batteries. Incorporating borate compounds into the solid electrolyte interphase (SEI) substantially decreases the decomposition reactions of the anode and cathode, greatly improving performance and safety. When applied to the anode or used in electrolytes, borates likely improve the surface interface, a critical layer that forms during cycling. When used to modify the surface of graphite, treatment with borates at less than 1000°C results in promising improvements in rate capability, life cycle, and capacity.
Where electrical capacitors devices that store electrical energy are concerned, borate chemicals are used in many formulations as well as in various parts of the manufacturing of aluminium-plate capacitors. And, boron-containing electrolytes are found in supercapacitors important energy devices that are enabling electric and hybrid electric vehicle technology.
Boron Improves Safety And Efficiency
- Lithium-ion batteries used in portable electronics use graphite as an anode material. However, graphite anodes are susceptible to lithium deposition and dendrite formation at high charge rates. These issues can short out the battery cell and cause safety issues. Borate surface coating may protect against lithium deposition, leading to better safety characteristics and potentially improving the stability of the SEI, which forms a boundary between electrode and electrolyte.
- High-temperature heat treatment of the graphite used in batteries contributes to a highly ordered crystalline graphite structure. But high-temperature graphitization is an expensive and energy-intensive process that requires temperatures in the 3000°C range. Adding borate before graphitization improves electrochemical properties and lowers the temperatures required for graphitization.
- In addition to increasing crystallinity, boron is thought to incorporate into the lattice structure of graphite at higher temperatures, initiating greater alignment and changing the electronic structure. In the graphite lattice, boron can act as an electron acceptor, leading to a specific capacity of 437 mAh/g higher than the theoretical maximum for pure graphite (372 mAh/g).