What is Electroanalysis
Electroanalysis is a process that determines the concentration of an analyte in a sample. This either measures the current or potential difference between two electrodes. Since boron can oxidize or reduce other substances in solution, it can offer superior performance, for instance using boron-doped diamond electrodes.
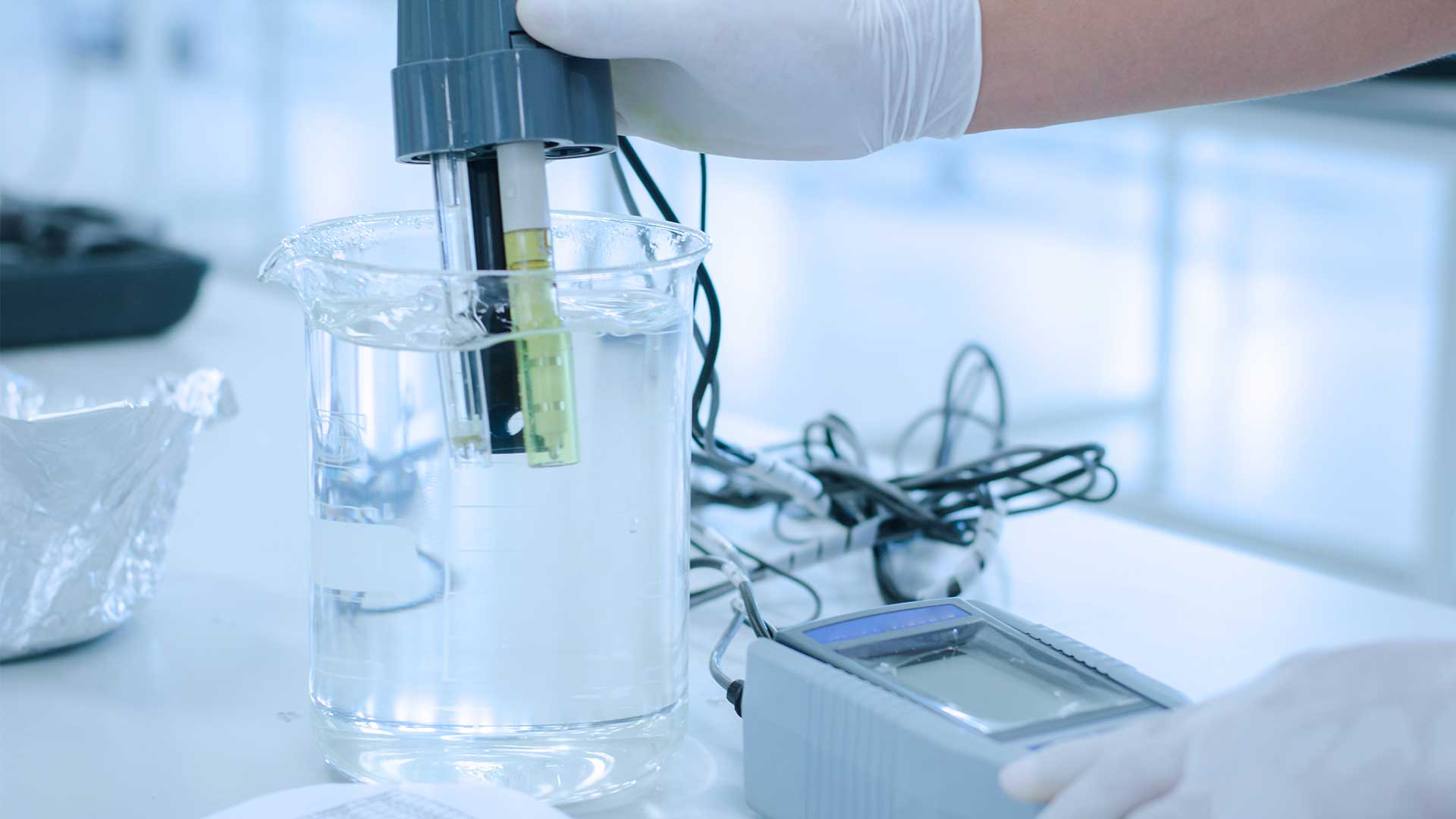
Electroanalysis with Boron
Electroanalysis is the second most crucial type of instrumental analysis. Electroanalytical methods contact the analyte solution using electrically conductive probes known as electrodes. The electrodes are used in combination with electric or electronic equipment to measure an electrical parameter of the solution. The monitored parameter is then linked with the analyte’s identity or quantity in the solution.
Classification of Electroanalysis
The electroanalytical methods are classified into five categories based on the monitored electric parameters: potentiometry, amperometry, electrogravimetry, voltammetry (and polarography), and coulometry.
Potentiometry Electroanalysis
This method determines the potential between two electrodes while controlling the electric current (usually near zero) between the electrodes. Potentiometry uses an indicator electrode whose potential fluctuates with analyte concentration and a reference electrode whose potential remains constant.
Potentiometry is the most commonly used electroanalytical method. It is classified into two types based on the nature of the indicator electrode.
When a metal or other conductive material is placed in the analyte, it demonstrates the potential of the bulk solution into which it is immersed. Platinum, gold, silver, graphite, and glassy carbon are common electrode materials for this type of potentiometry.
Amperometry Electroanalysis
Amperometry is an electrochemical technique that measures the concentration of an electroactive species in a solution. The potential of the indicator electrode is corrected to a value on the voltammetric wave’s plateau, and the resulting current is measured.
This current is directly proportional to the concentration of the analyte.
Amperometry can be used in two ways, both of which benefit from the current linear variation at the constant potential of an electroactive species concentration.
In an amperometric titration, a working current as a function of the concentration of standard solutions is prepared, and the analyte concentration is measured from the curve. A current graph as a function of titrant volume is an amperometric titration curve. The curve shape changes according to the electroactive chemical species (titrant, analyte, or reaction product). The curve in each case is made up of linear regions before and after the endpoint is extended to overlap.
Electrogravimetry Electroanalysis
Electrogravimetry, like voltammetry, makes use of two or three electrodes. A constant current or a constant potential is applied to the preweighed working (indicator) electrode. When applying an electric current or potential, a solid product of the analyte’s electrochemical reaction protects the electrode. The working electrode is removed, rinsed, dried, and weighed after the tested substance has been entirely removed from the solution by the electrochemical process. The increased electrode mass caused by the presence of the reaction product is used to calculate the analyte’s initial concentration.
Coulometry Electroanalysis
Coulometry is used in either constant-current or constant-potential modes to determine the quantity of an analyte in a sample by measuring the amount of electricity required to complete its electrochemical reaction.
This makes it possible to assay for substances that do not deposit on electrodes, as long as the current flowing through the electrode is used for a single electrochemical reaction. This requirement is met in constant-current coulometry by performing a coulometric titration with the current.
The current in a coulometric titration produces a titrant that reacts chemically with the analyte. This ensures that only one electrochemical reaction occurs and that all of the current produced by the reaction is used to form the titrant. The result is a quantitative analysis that is immune to interference effects.
Voltammetry Electroanalysis
Voltammetry analyzes a wide range of molecular and ionic materials, qualitatively and quantitatively. In this method, a two or three-electrode set is immersed into the analyte solution, and a varying potential is applied to the indicator electrode concerning the reference electrode.
At the indicator electrode, the analyte electrochemically reacts. The reference electrode is used to maintain a constant potential irrespective of the solution into which it is immersed.
Typically, a third electrode (an auxiliary or counter electrode) is put in the solution to carry most of the current. The potential is managed between the indicator and reference electrodes, but the current flow between the auxiliary and indicator electrodes.
Classic Polarography Electroanalysis
Polarography is voltammetry with mercury or, in rare cases, another liquid metal indicator electrode. In classic polarography, mercury drops from a capillary tube onto the surface of the electrode, where the electrochemical reaction with the analyte takes place.
In a potential (or voltage) ramp, the indicator electrode’s direct current (DC) potential varies over time. When the potential varies linearly with time, the analytical method is linear sweep voltammetry (LSV).
Triangular Wave Voltammetry Electroanalysis
Triangular Wave Voltammetry is a method in which the potential is uniformly scanned to a value beyond the potential at which an electrochemical reaction occurs and then instantly scanned back to its original potential. A triangular wave voltammogram typically has a forward current peak and an inverted peak on the reverse scan indicating the opposite reaction (oxidation or reduction) to that reported on the forward scan. Cyclic voltammetry is similar to TWV, but it performs more than one forward cycle and reverses scans in a row.
AC Voltammetry Electroanalysis
AC voltammetry helps to study the reversibility of electrochemical reactions by forcing the reaction in the forward and reverse directions. By measuring only the AC portion of the current, we can get a clear picture of how reversible the reaction is.
In AC Voltammetry, an alternating potential is plotted to the DC potential ramp for LSV.
Pulse and Differential Pulse Voltammetry Electroanalysis
The voltage ramp in linear sweep voltammetry is plotted with a regularly applied potential pulse in differential pulse voltammetry.
The current is measured before and after the pulse is applied. The difference in currents between the two is plotted as a function of the LSV ramp potential.
Pulse voltammetry employs a continuously increasing pulse height applied at regular intervals. Pulses are used before the mercury drop falls from the electrode. The pulse is typically used for 50–60 milliseconds, and the current is recorded during the final 17 milliseconds of each pulse. The voltammogram is a plot of the measured current versus potential.
Applications of Boron-doped Diamond Electrodes In Electroanalysis
The development of BDD electrodes has been driven by the need for more sensitive and stable detection of various substances. These electrodes have excellent electroanalysis properties, such as a broad potential window, low background current, electrochemical stability, and fouling resistance. As a result, they can be used for drug detection, bio-related substance detection, metal ion detection, and organic pollutant detection. Many articles have reported successful detection of actual samples using BDD electrodes, demonstrating their utility and practicality in various applications.
The surface of the BDD electrodes can be modified with metal nanoparticles, nano carbons, and polymers to increase the sensitivity of electrochemical detection.
BDD surface termination, the effect of boron doping level, and the use of analyte adsorption on BDD surfaces for the development of adsorptive voltammetric techniques are discussed.
Furthermore, research on electroanalytical devices equipped with BDD electrodes will be expanded by combining peripheral device fabrication technologies.