Introduction to Boron Thermal Properties
Boron thermal properties are influenced by temperature and radiation. The trigonal structure of Boron is described by the unit cell. Lattice Angles are the positions of the atoms within the unit cell. Its molecular weight determines the atomic positions of a substance. The molecular weight of a material is related to its temperature properties. This means that Boron has a high heat capacity.
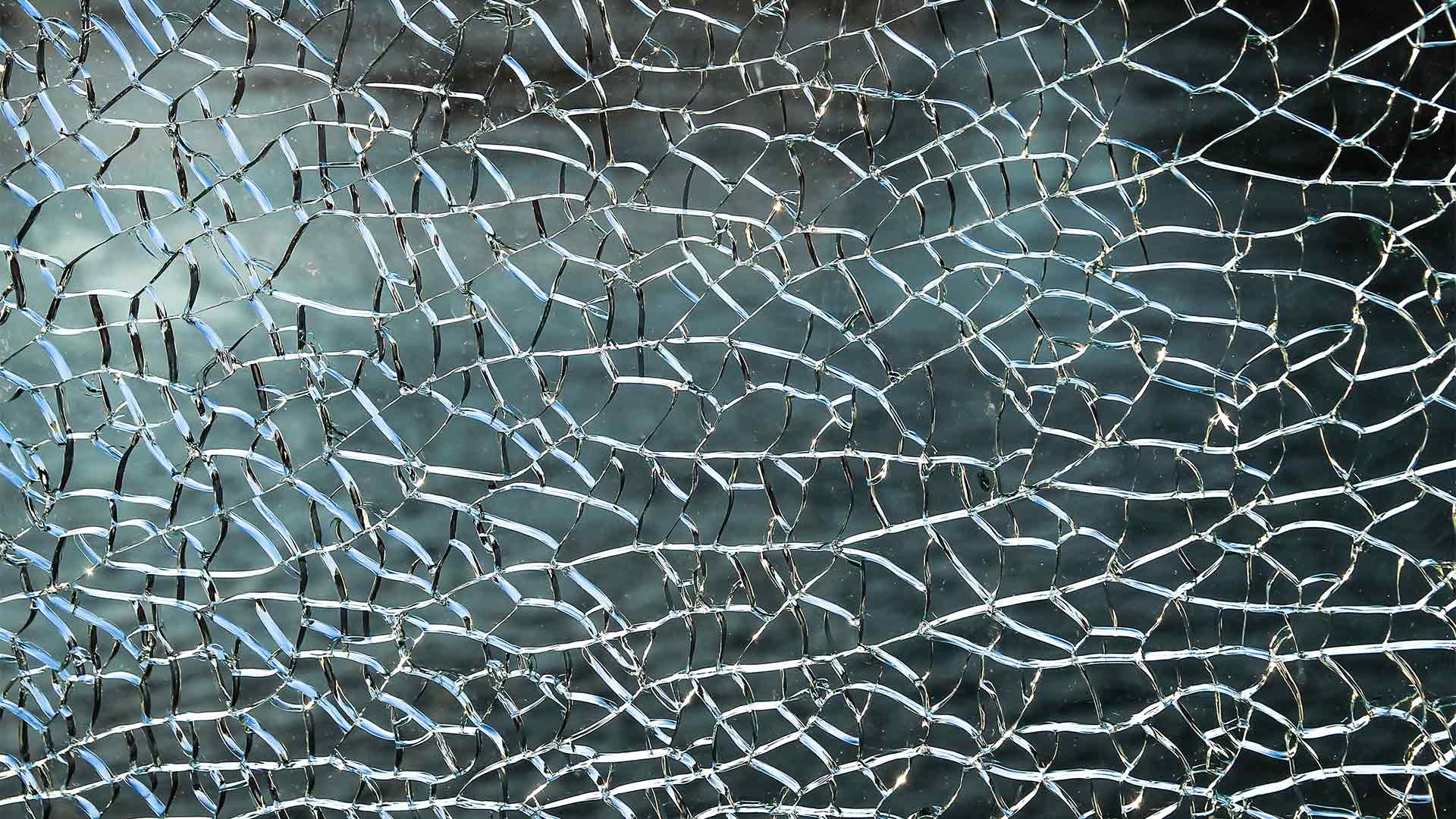
Boron Thermal Properties
The melting and boiling point of Boron is 2079 and 3927 degrees C, respectively. These values are linked to standard atmospheric pressure. Amorphous boron nitride is a good window treatment insulator. However, the thermal conductivity of a single molecule of Boron is too low to be manipulated. To manipulate thermal conductivity, it must be combined with a cell. Once it has been processed, it gains a higher thermal conductivity. The process also improves efficiency.
Table: Thermal Properties of Boron
Thermal Property | Value |
---|---|
Melting Point [Celsius Scale] | 2079 |
Boiling Point [Celsius scale] | 2550 |
Thermal Conductivity [W/m K] | 27 |
Specific Heat [J/g K] | 1.02 |
The heat of Fusion [kJ/mol] | 50.2 |
The heat of Vaporization [kJ/mol] | 489.7 |
Source – Material properties.org
Borosilicate melts and boils at temperatures of 2,079 and 3,927 degrees Celsius, respectively, when under standard atmospheric pressure. The thermal conductivity is measured in watts per meter of kelvin. The values are the same for all matters. The higher the value of a substance, the greater its thermal conductivity. At higher temperatures, heat is transferred more readily. The material will become unstable if it is not conducive.
The thermal conductivity of Boron is similar to that of aluminum and copper. The thermal conductivity of Boron is 27 W/(m·K). Its electrical conductivity and heat energy are the same. However, compared to aluminum, Boron is a minimal quantity of material, making it less suitable for high-temperature applications. In addition, it is not as strong as copper or iron, so it will be difficult to use. In contrast, tungsten is a relatively dense metal.
Its thermal conductivity characterizes the chemical properties of Boron. The solids in the earth’s crust have high thermal conductivity. For example, Boron contains more than one million times more energy than its neighbor aluminum. This means that it is highly reactive, making Boron a good candidate for using it in electrical applications.
The thermal conductivity of Boron is essential for various reasons, from insulating materials to acoustic insulation. The linear thermal expansion coefficient of Boron is 5-7 µm/(m·K). As a consequence of a temperature change, matter expands in size. As a result of the temperature change, it is usually expressed as a fractional change in length or volume.
The Solid Boron Nitride grade HP is a superior grade for thermal applications at 1,000degC. It has ten times the moisture resistance of Grade A. It is used in a variety of light metal and electrical applications. This conductive material is highly resistant to corrosion, so it is a good choice for many applications. Its thermal conductivity is an essential factor for determining the life expectancy of a product.
Its thermochromic nature also allows it to be a good conductor of electricity and heat. For electrical applications, it is a good insulator. Its low thermal resistance makes Boron a good candidate for capacitors. The same properties make it a good candidate for electronics. If you’re looking for an electrical insulator, boron nitride is an excellent choice. This material has the following advantages:
PCBN4000 is a higher-temperature grade of hexagonal Boron Nitride. This material is accessible to machines and has good thermal shock and thermal conductivity. It is also used in many other types of electronic equipment, including in high-temperature devices. It is used in various applications for its excellent properties. It is possible to manufacture high-end appliances utilizing this material when it comes to electrical devices. It also works well with batteries.
HP-BN4000 is a workhorse grade of boron nitride. Its big thermal shock and amorphous carbon are its other benefits. Thermoelectricity is one of the best thermoelectric properties of Boron. Its amorphous carbon does not absorb and retain heat well. As a result, it can produce a variety of thermoelectric devices. The material is solid.
Unlike other materials, boron nitride is an excellent heat conductor. Its high-temperature range and low dielectric constant make it suitable for high-temperature insulators and light metal applications. Both grades are easily machined and can be machined into complex shapes. A Grade is the most common boron nitride available and is an excellent choice for many applications. There are several types of Boron nitride available.
Case Study: Stability of standard state boron structure revealed
Angewandte Chemie published a paper on January 23, 2015, titled “Determination of Phase Stability of Elemental Boron,” demonstrating Boron’s stable phase at room temperature. Before this work, two structures, ???? Boron and ???? Boron, were considered contenders, but neither one was considered more stable.
Study Findings
A chemical thermodynamic cycle was used to conduct the majority of the research. Compared with their parallel studies of β-boron, we produced a sample of α-boron and calculated its thermodynamic properties with great accuracy.
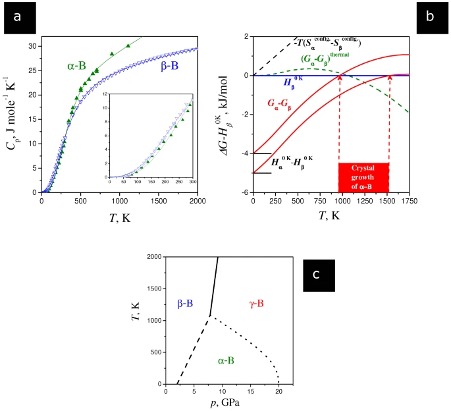
Boron Thermal Properties
Graph Explanation
(a) Measure the heat capacity of compounds α-B12 and β-B106 at ambient pressure. Solid lines represent the Holzapfel equation54,55, while triangles represent experimental data56,57,58.
(b)Calculation of the formation enthalpy (Hα0K − Hβ0K) of α-B12 at ambient pressure (β-B106 was considered as a normal state). It is shown in black dashed lines that there is a difference between the Gibbs energies of α-B12 and β-B106 due to the non-zero configurational entropy of β-phase, i.e., T Sβ0K (Sα0K = 0, Sβ0K ~ 0.5R)49. Green dashed lines depict the difference between thermal contributions (due to the differences in heat capacities) into the Gibbs energy for α-B12 and β-B106.
Blue solid lines indicate the zero energy level chosen as Hβ0K. By comparing the highest and lowest temperatures at which α-B12 crystals can be synthesized, one can estimate that Tα–β should be between 933 K50 and 1500 K51 (red rectangle area). Two red curves depict two different Gibbs energies of α-B12 (Gα − Gβ) for two different values of (Hα0K − Hβ0K); while these enthalpy values give the temperature limits (933 K50 and 1500 K51) for the α β equilibrium (Gα(T) − Gβ(T) = 0). Therefore, the average value of (Hα0K − Hβ0K) is about − 4.5 kJ/mol when crystallization of α = B12 occurs at equilibrium conditions between these two temperatures.
(c) A proposed low-temperature phase diagram for Boron. Similar to the previous simulations1,10, the α β equilibrium line (dashed) crosses the pressure axis. The slope (dp/dT)α–β = ΔSα–β/ΔVα–β is positive and has a non-zero value at 0 K due to the configurational entropy of β-B106. The (dp/dT)α–γ → 0 when T → 0; and at low temperatures, the α γ equilibrium line (doted) is parallel to the temperature axis, while at a higher temperature it joins the triple point defined by the intersection of the calculated α β (dashed) and experimental β γ (solid) equilibrium lines.
Source – Nature equilibrium
In the presence of ambient pressure, β-boron is found to be the most stable structure, up to a temperature of 2000 k. The higher stability of β-boron is surprising since β-boron atoms are less dense, and the lattice positions for these atoms are partially occupied. This indicates that the entropy of β‑Boron is not zero at T = 0 K. This residual entropy property is not found in any other element.
The thermodynamic analysis has shown that β-boron is entropically stabilized concerning α-boron at temperatures below 1000 K due to its partially occupied sites. Due to substantial differences in the heat capacities of the two types of Boron, β-boron is enthalpically stabilized compared to α-boron at higher temperatures.
We have also demonstrated that α-boron only becomes more stable than β-boron under pressure thanks to Tadashi Ogitsu at Lawrence Livermore National Lab.
Brief Background
From different perspectives, Mark Tuckerman discusses the proton, chemical bonds, and first-principles calculations. On earth, Boron does not occur in its pure elemental form. However, it is found in many beneficial compounds, such as bulletproof vests, powerful tools, and aerospace technology. Borosilicate glass is the second hardest material on earth, followed by boron carbide.
Diamond is the most complex material. However, boron compounds can be used at high temperatures where diamond burns. In addition to being used as abrasives, tank armor, and nozzles for sandblasting, these boron-containing materials can also be used for tool bits.
Borosilicate glass can be heated and cooled without cracking, as it has a very low coefficient of thermal expansion; it is often marketed as Pyrex. Nanotubes made from boron nitride promise aerospace applications, thanks to their strength, lightweight, and radiation shielding properties.
Surprisingly, magnesium diboride is a superconductor. Other borides are being investigated as thermoelectric materials that convert waste heat into electricity. To make more accurate predictions about the properties of boron-containing materials, we must understand the fundamental properties of Boron.